PK Effect on Pre-Recorded Targets
HELMUT SCHMIDT
(Originally published in The Journal for the American
Society for Psychical Research Vol.70, July 1976)
ABSTRACT: In three PK experiments the random events to be affected were
generated and recorded in the absence of both subject and experimenter.
The subject became involved only later when the pre-recorded events were
played back to him.
The first experiment used an electronic random number generator with hit
probability = 1/64. In the first part of the test the hits were displayed
immediately as weak clicks on which the subject had to concentrate. In
the second part the clicks were first recorded on magnetic tape and later
played back to the subject. In both cases an increase in click rates above
chance expectancy was observed (P=.001).
In the second experiment loud clicks were randomly (hit probability = 1/2)
channeled to the right or left ear while the subject tried to enforce an
increased click rate at the right ear. Half of these events were
momentarily generated while the other half came from a replay of an
earlier recording. Each recording was used for four sessions so that
subjects spent four times as much effort on each pre-recorded event than
they did on each momentarily generated event. The scoring rates on the
pre-recorded and momentarily generated targets were 52.9% and 50.8%
(P=.005 and .05, respectively). This confirms the existence of a PK
effect on pre-recorded targets and suggests that repeated replay of the same
targets may lead to higher scoring rates.
In the third experiment the task was the same as in the previous one, but
the binary events came from an "easy" generator with a hit probability of
7/8 and a "difficult" generator with a hit probability of 1/8. A
pre-recorded sequence of +1's and -1's determined the order in which the
easy (+1) and difficult (-1) generators were activated. The subject was
successful if in this sequence he obtained an excess of +1's, and in
addition he could improve his score by affecting the easy and difficult
generators directly. The results were not significant enough to permit
a detailed comparison between the direct PK effect and the PK effect on
the pre-recorded sequence, but the latter was confirmed at the .05 level.
INTRODUCTION
Consider the following experiment: A random number generator is activated to produce a string
of N binary numbers. These numbers are automatically recorded on magnetic tape, paper punch
tape, or some other reliable recording medium. Nobody is present during this generation and
recording, and nobody looks at the data until at some
later time the recorded sequence of "heads" and "tails" is played back to a subject in a PK test
situation. During the slow playback each recorded head or tail makes a red or green lamp light
up while the subject tries mentally to enforce an increased lighting rate of the red lamp.
One might think that in this situation the subject could not succeed because the decision as to
how many heads and tails will appear has already been made before the test session. But one can
also present arguments that PK might still operate, and that, furthermore, such PK tests with time
displacement could give some interesting new insights into the physics and psychology of psi.
Let us look at some of these arguments.
A Mechanism of Subconscious Goal-Oriented PK
Many earlier studies (e.g., Schmidt, 1974; Stanford, 1974) suggest that PK is goal-oriented and
is largely activated by subconscious factors. Therefore, in the hypothetical experiment mentioned
above, the subject and/or the experimenter might subconsciously initiate a PK mechanism at the
time when the binary numbers are generated. And this could lead to a successful outcome of the
test. In this interpretation, a PK effect on prerecorded targets could occur even if PK acts in a
causal manner like the other known forces of nature; i.e., we would not have to assume for PK
any of the features of time independence which are familiar to us in the realm of ESP.
From this viewpoint it would be interesting to measure in the experiment the subject's and the
experimenter's mood (or some other physiological-psychological parameter) at the time of target
generation and also at the time of the test session to see how these measures correlate with the
PK score.
Possibility of a Noncausal PK Mechanism
When the time independence of telepathy and clairvoyance became apparent, i.e., when the
reality of precognition was established, this seemed quite contrary to common sense. People
asked by what "mechanism" the outcome of a future random event could cause something (the
subject's correct response) to occur in the present. But in spite of this conceptual difficulty,
precognition continues to exist, and rather than wondering what is wrong with precognition, we
should ask what is wrong with our common sense.
The fault with this "common sense" is that it is based on the picture of a
causal world in which a cause may produce some later effect,
but not vice versa. This picture seems applicable to the vast majority of phenomena
observed in everyday life and in the physics laboratory, but not to precognition.
If ESP is noncausal in the sense that a future random event may produce an earlier effect (the
subject's correct response), we may have to consider the possibility that PK is noncausal in the
sense that a present random process may be affected by the subject's PK effort made at some
future time. In view of the close relationship between PK and ESP (Schmidt and Pantas, 1972),
one might even be surprised if PK did not share the feature of time independence with ESP
phenomena.
Implications of a Theoretical Psi Model
Recently a mathematical model of psi was discussed (Schmidt, 1975) in which precognition, PK,
and other forms of psi appear as outcomes of one unifying psi principle. This model may be too
simplistic, but it is logically self-consistent and thus permits us a full discussion of those vexing
aspects of psi which seem to be contrary to common sense.
This model predicts in particular that the score in a PK test with time displacement should be
essentially the same as the score in a corresponding conventional PK test. The outcome of the
test should depend only on the test conditions (such as mood of subject and experimenter, nature
of feedback, etc.) at the time of playback, but not on the conditions at the time when the targets
were generated. A quite specific and easily testable feature of this model is, furthermore, that
the repeated playback of a prerecorded target sequence to the subject should lead to an increase
of the PK scoring rate.
Possible Connection with the Reality Problem of Quantum Theory
No development of modern science has had a more profound impact on human thinking than the
advent of quantum mechanics. Wrenched out of centuries-old thought patterns, physicists of a
generation ago found themselves compelled to embrace a new metaphysics. The distress which
this reorientation caused continues to the present day. Basically, physicists have suffered a
severe loss: their hold on reality (Bryce S. DeWitt, 1971).
In the hypothetical experiment mentioned above, it seems intuitively obvious at which time the
random decisions are made: when the generator is activated and the outcome (head or tail) is
recorded. The experimental findings of quantum physics raise some doubts, however, whether
this common-sense judgment is meaningful. We may see the origin of the problem by
considering first the special random number generator shown in Figure 1.
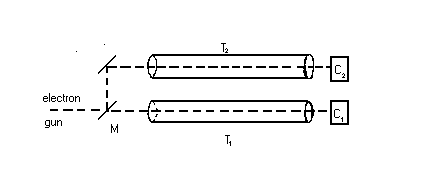
Fig.1. A binary random number generator built from an electron gun, a
semitransparent mirror (M) and two electron counters.
This generator consists of an electron gun which emits, say, every minute one electron toward
a semitransparent mirror M, so that each electron has a 50% chance of penetrating the mirror and
entering the
tube T1 and a 50% chance of being reflected into the tube T2 If we install electron counters C1,
C2 at the end of these heavy metal tubes T1, T2, then, under somewhat idealized conditions, each
arriving electron will be registered by one of the counters. It will never happen that an electron
gets split into two parts so that each of the counters registers half an electron, because no half
electrons exist in nature. Thus we have an ideal binary random generator: every minute one of
the two counters registers an electron and the decision which counter will respond next is made
by an elementary quantum process which should be determined by pure chance.
It may appear intuitively obvious that the random generator has made a decision whenever an
electron has passed the mirror M and reached the entrance to the tubes. This is because an
electron which has entered, say, T1, cannot escape through the wall of the tube and is thus bound
to activate the counter C1. But this argument appears inconsistent with another experiment:
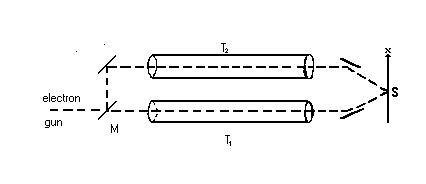
Fig.2. Setup for an electron interference experiment.
Suppose we remove the counters C1 and C2 and let the emerging electron beams converge on
a screen S (see Figure 2), and we then
measure with a large number of electron counters where each electron arrives at the screen. If
we continue the experiment for many hours, emitting one electron per minute, then we can map
the average particle arrival density for each point of the screen. The experiment shows that the
electrons arrive over a spread-out area and
that the arrival density fluctuates along the X-axis as indicated by Figure 3a. This pattern can be
interpreted only if we consider each electron as a wave which traveled through both tubes
simultaneously and thus produced an interference pattern at the screen. This interpretation is
supported by the fact that the closing of either one of the tubes changes the arrival density to a
smooth distribution indicated by Figure 3b.
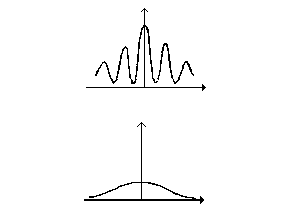
Fig. 3a (above) Electron arrival density in the interference
experiment.
Fig. 3b. (below) Electron arrival density if on e of the two tubes is blocked
Thus in the arrangements of Figures 1 and 2 respectively, the electrons seem to behave differently
when they reach the tubes. It appears that in Figure 1 each electron enters only one of the tubes
while in Figure 2 it travels as a wave through both tubes simultaneously. The odd feature of this
result is that an electron, at reaching the entrance of the tubes, should not yet "know" whether it
will encounter the arrangement of Figure 1 or Figure 2. Indeed, the experimenter might even
change from one setup to the other while the electron is already inside the tubes.
The parapsychologist might feel tempted at this stage to ascribe some "precognitive features" to
the electron, but in extensive experimental and theoretical studies over the last four decades
physicists have found no indication that the unusual behavior of the electron could be utilized
to predict the future in the manner human subjects can predict future random events. Quantum
theory has coped with the paradox successfully, not by invoking some noncausal mechanism, but
by a change in the concept of "physical reality."
In the arrangement of Figure 1 we feel intuitively that after the electron has reached the tubes it
must be "really" in one or the other tube. But in the quantum mechanical formalism the physical
state of the system is at this stage a "superposition" of two possible states, one state with the
electron in T1 and one state with the electron in T2. Nature has not yet decided for one of these
two possibilities, and that is why we can still shift to the test arrangement of Figure 2 and observe
an interference effect between two states. The decision as to which one of the two possibilities
is "real" occurs when the experimenter makes a measurement with the electron counters and finds
the electron in one or the other tube. It appears unreasonable to even talk about physical reality
(in the sense that the electron must be really" here or there) before the experimenter has made
his observation (to find out where the electron is). "Reality" is only what the experimenter
observes.
The loss of a physical reality which exists independently of the observer as an intuitively obvious
concept may not be restricted to small systems like single electrons, but may carry over into the
macroscopic world: assume that in the experiment of Figure 1 the counters are connected to an
automatic punch tape recorder so that each electron received by C1 or C2 is recorded as a hole
on either the right or the left side of a paper tape. Then, after the electron has reached a counter,
but before an observer has taken notice of the results, the current state of the system is described
by quantum theory as a superposition of two possible states: one state where the counter C1 has
recorded a particle and a hole is punched on the right side of the tape, and one state where the
counter C2 has recorded the electron and a hole is punched on the left side of the tape. These two
states are coexistent; nature has not yet decided for one or the other. The decision is made when
the experimenter takes notice of the results.
Many attempts have been made to modify or to re-interpret quantum theory so that the concept
of an absolute reality could be saved at least at the macroscopic level. But with the present tools
of physics we have no means of testing whether the outcome of a random process becomes
finally decided when the result is macroscopically recorded or when the human observer takes
notice of the results.
With the tools of the PK tests we might have another access to the reality problem. We might
tentatively assume (somewhat naively, in view of the noncausal aspects of PK) that a present PK
effort can only affect random processes of which the outcome is not yet decided. (We could even
try to define physical reality by the requirement that the state of a physically real system cannot
be changed by PK efforts.)
From this viewpoint the comparison of PK on random processes before or after they have been
macroscopically recorded (i.e., the
comparison between conventional PK and PK on prerecorded targets) seems particularly
interesting. If the described interpretation of quantum theory is right, then the PK effort after the
recording should be equally successful, because at this stage nature has not yet reached a decision
on the outcome. Thus the PK effect on prerecorded targets appears as a natural consequence of
the conventional PK effect. An interesting modification of the experiment results if the PK effort
is made after some human experimenter has looked at the outcome. This latter case is not
studied, however, in the present report.
THE FIRST EXPLORATORY STUDIES
The first encouraging PK result with prerecorded targets was obtained by the present writer in
1971 at the Institute for Parapsychology in Durham, North Carolina, with Mr. Lalsingh
Harribance as subject.
As a first step in this experiment, a fast binary random generator was automatically activated to
generate many blocks of 201 binary numbers. The generation rate was 20 numbers per second
and the blocks were separated by 20-second intervals. The "heads" and "tails" were registered
as clicks in the right and left channels of a stereo cassette recorder. In this manner six "primary"
tapes were prepared with an average of about 140 blocks per tape.
As a next step the first three primary tapes were copied on one-channel tapes so that each head
or tail appeared on these "secondary" tapes as a weak or a strong click, respectively. They were
then given to the subject to take home. He was instructed to play each tape once, to listen
carefully to the weak clicks (while ignoring the strong clicks as far as possible), and to enforce
an increased occurrence of weak clicks. The subject was encouraged to spread the test over many
days and to take frequent breaks.
The heads and tails on the corresponding primary tape were electronically counted only after the
subject had listened to a whole tape. The recovery of all clicks could be verified since the total
number of clicks in each block was known.
In order to evaluate the results, the number of successful runs (block with more heads than tails)
and the number of unsuccessful runs (block with more tails than heads) were counted. A total
of 236 successful runs and only 189 unsuccessful runs were found, which is suggestive (CR =
2.38) of a PK effect on the prerecorded targets. Note that the subject never got in touch with the
primary tapes on which the evaluation was based.
This type of experiment, where the subject works at home without
the presence of an experimenter, might have many psychological advantages. It might provide
a convenient means of channeling psi effects from the quietness of the subject's living room into
the laboratory.
The other three prerecorded primary tapes were played back to the subject directly in the
laboratory. Feedback was given by an instrument needle which was moved by each head or tail
(as they were replayed from the tape) one millimeter to the right or to the left, respectively, while
the subject tried to make the needle move to the right. The result of this part of the experiment
was 218 successful runs and 189 unsuccessful runs (CR = 1.44). The total experiment gave a CR
of 2.71 in favor of the existence of PK with pre-recorded targets.
The experiment had to be discontinued for technical reasons, but in 1972 some other pilot tests
were carried out. Two of these studies, with Mr. Lee Pantas and Dr. E. F. Kelly, respectively,
as experimenters, again gave encouraging results.
In these studies a four-choice random generator was used. The generator was automatically
activated to generate a long random sequence of the numbers 1, 2, 3, and 4. This sequence was
stored on paper punch tape. Nobody looked at the data until these numbers were read back in
the test session by an electromechanical paper tape reader.
During the test session the subject sat in front of a panel with four lamps. Whenever he pressed
a button the tape reader read the next number on the tape and made the corresponding lamp light.
The subject's task was to make the lamp corresponding to the number 4 light with increased
frequency. Thus he could succeed only if the prerecorded tape contained an increased number
of 4's. The subject's score was automatically registered during the test, and could also be verified
by another replay of the tape.
In his experiment Mr. Pantas compared the score of one subject, M. S., who had been particularly
successful in previous tests, with the score of an unselected group of subjects. In both cases the
targets came from the same long tape of prerecorded numbers. The 4100 trials made with M.S.
gave a hit deviation of +72 (CR = 2.6), whereas the unselected croup in 4700 trials scored near
chance (deviation of -7). After the completion of the experiment the unused part of the tape was
evaluated by computer and showed no significantly increased number of 4's (deviation of +20
in 52,730 trials). Thus only the part of the tape which was used in the PK test with the special
subject showed a significantly increased occurrence of 4's.
Dr. Kelly used the same test arrangement, but he had only one subject, Mr. Bill Delmore, who
has obtained outstanding scores in experiments with different researchers. In a total of 8930
trials the
deviation in the number of hits from chance expectation was + 158 (CR = 3.86).
These experiments are presented only as suggestive pilot studies, and it is not possible to assess
the significance of the results rigorously because we do not have complete records of similar
experiments (which were mostly aborted at an early stage) which did not produce positive scores.
The three experiments to be reported below, however, form a complete record of all confirmatory
PK studies with prerecorded targets performed so far by the writer. Each of these confirmatory
studies was begun after a previous pilot test had indicated that the conditions for observing PK
were favorable in the given psychological-physical setup. The length of each confirmatory study
and the main method of data evaluation were specified in advance.
THE FIRST EXPERIMENT: PK EFFECT ON A RANDOM TIME INTERVAL,
WITH AND WITHOUT TIME DISPLACEMENT
This study was begun as a conventional PK experiment. After a
pilot test and a confirmatory series had given significant results, a
second series of tests was added, with the only difference being that
the PK targets were recorded in advance of the test situation.
The Conventional Part of the PK Test
Let us consider first the conventional part of the experiment. In this experiment an electronic
random generator with p = 1/64 and q = 63/64 was used. For each test run the generator was
automatically activated, at the rate of 10 trials per second, until a hit (p = 1/64) was obtained.
Then the generator stopped and the hit was displayed to the subject through headphones as a
weak click.
A display counter advanced with each trial made so that the
reading of the counter at the end of a run gave the number R of trials
which had been required for obtaining a hit. The chance expectancy
for R is R~ = 64 (corresponding to an average run length of 6.4 seconds) and the chance probability
for a particular R to occur is easily seen to be P(R) = p*q^(R-1), with p = 1/64, q = 63/64.
In this experiment an attempt was made to guide the subjects' PK efforts at a subconscious level
such as to increase the hit probability; i.e., to reduce the average length of a run. The subjects
were not aware of this goal but they were instructed to listen very carefully to the barely audible
clicks so that they would not miss any of the clicks terminating a run. The challenging aspect
of the task was strongly emphasized to the subjects and they were encouraged to associate the
task with some realistic life situation. Thus, for example, a subject might imagine himself in a
forest, listening to faint bird sounds.
It was hypothesized that the subjects' eager and expectant concentration on the next click would
activate a PK mechanism such as to make this click come in earlier than expected by pure
chance.
At the beginning of each session the sound volume was individually adjusted so that the subject
could hear approximately 80% of randomly presented clicks. Then 20 runs were made with a
break of typically 10 minutes after 10 runs. Some minor psychological differences in the test
situation for the pilot test, the confirmatory study, and the test with time displacement are
discussed in the following.
The pilot experiment. After some preliminary, informal tests a pilot study was begun in
December, 1973, at the Institute for Parapsychology. This study consisted of 20 sessions with
20 different subjects who were members of the laboratory and visitors who expressed interest in
this particular experiment.
During a test session the subject, fitted with headphones, was seated in a comfortable chair and
was encouraged to relax. No attempt was made to shield the room against external noise. For
each test run the experimenter gave a signal to the subject and then started the random generator.
When the run ended the weak click was presented to the subject and the advancing display
counter in front of the experimenter stopped.
In this pilot test the experimenter was more personally involved than in the later tests in two
respects:
1. At the end of each run the experimenter manually recorded the counter reading.
2. The experimenter gave a start signal to the subject at the beginning of each run, and a stop
signal in the cases where the subject had not noticed the click at the end of the run. Thus the
experimenter had to check the display counter occasionally to see whether the run was still in
progress.
For the discussion of the results let us call each decision made by the random generator a "trial."
Then in a run the generator makes a certain number R. of consecutive trials until a hit (p = 1/64)
is obtained and the run ends. Thus each run contains one hit in a varying number R of trials
which is given by the display counter.
For the pilot study 20 different subjects contributed 20 runs each so that the total number of hits
(H = 400) was pre-specified, rather than the total number N of trials. Nevertheless, it is easily
seen that we can still derive the statistical significance of the result in the usual manner from CR
= (H - Np)/((Npq)^(1/2)). The results given in Table 1 (Column 1) indicate that the average run length
was significantly reduced from the chance expectancy of 64 to the observed value of 54.23. At
the trial rate of 10 per second, this implies that the average waiting time for the next click was
reduced from 6.40 to 5.42 seconds.
Table 1
MAIN RESULTS OF THE FIRST EXPERIMENT
| Conventional PK | Test with Pre-Recorded Targets |
| I: Pilot | II: Conf. | III: Test Runs | IV: Controls |
Number of Subjects | 20 | 30 | 30 | --- |
Number of Trials N | 21693 | 33205 | 33819 | 37864 |
Number of Hits H | 400 | 600 | 600 | 600 |
Average Run Length N/H | 54.23 | 55.34 | 56.37 | 63.08 |
CR | 3.34 | 3.59 | 3.14 | .40 |
t (19 or 29 df) | 3.29 | 3.62 | 3.66 | .50 |
P (one-tailed) | .001 | .001 | .001 | n.s. |
Note. The chance hit probability is p = 1/64 and the average run length, the average
number of trials required to obtain a hit, is 1/p = 64.00.
In addition to the CR value for a group of subjects, Table 1 also gives the t-values based on the
contributions of the individual subjects. Both these values, CR and t, provide valid measures for
the existence of some PK effect in the group. If, furthermore, the contributions of the group
members were independent, we could draw from the t-value further conclusions about the
distribution of PK abilities over the population, and this would permit statistical predictions
about the outcome of later similar experiments.
This requirement of independence, however, need not be satisfied in psi tests because a subject's
psi performance does not (like his height or weight) depend exclusively on the subject, but is
co-determined by other systematic factors, for example, the mood of the experimenter. Thus, if
some of the subjects are tested while the experimenter is in a "good" mood and the others while
the experimenter is in a "bad" mood, there may be a systematic difference between the two
sub-groups, violating the assumption of subject independence. Therefore we must be cautious
if we want to derive from a t-value anything more than the operation of some psi effect.
Extensive randomness tests carried out between experimental sessions verified the value 64 for
the chance expectancy of the run length when no PK effort was applied (4000 such runs gave an
average run length of 64.48, which is consistent with the assumption of the ideal operation of the
random generator). Thus the abnormal scoring in the test sessions appears to be a PK effect.
One might want to interpret this effect tentatively in the sense that
the subjects in their eager concentration on the next click had subconsciously activated a PK
mechanism to make this click come in earlier than expected by chance. But there are certainly
other possible interpretations: the subjects might have directed their PK effort such as to please
the experimenter (i.e., to confirm his expectation), or the experimenter might have activated his
own PK mechanism for this purpose.
Table 2
DISTRIBUTION OF THE OBSERVED RUN LENGTHS R IN THE
FIRST EXPERIMENT
| Conventional PK |
Test with Pre-Recorded Targets |
Interval for R | Associated Probability | I: Pilot |
II: Conf. | III: Test Runs | IV: controls |
1-14 | .1979 | 98 | 129 | 145 | 119 |
15-32 | .1980 | 80 | 143 | 126 | 120 |
33-58 | .2030 | 87 | 131 | 128 | 114 |
59-102 | .2005 | 76 | 107 | 105 | 124 |
103- | .2006 | 59 | 90 | 96 | 123 |
Note. The five intervals are chosen so that the associated chance probabilities for a run length
R to fall into any one interval are approximately equal. The table gives the observed number of
events in each interval.
The trend toward a reduction of the average run length in the experiment is
also reflected in the data of Table 2 (Column I). There
we have divided the R-axis into five intervals which should by chance contain approximately
equal numbers of events. The increased number of events in the intervals corresponding to
shorter runs is seen. The interval 1 <=R<=14, for example, contains 98 events (chance
expectancy 79.2), whereas the interval 103<=R < infinity contains only 59 events (chance expectancy
80.2). This difference alone is significant (CR = 3.16).
The confirmatory experiment. After the completion of the pilot study a confirmatory test was
begun and finished in January, 1974. The pre-specified number of 30 subjects contributed one
session each. The subjects were visitors to the laboratory who had expressed interest in the
particular experiment.
In an attempt to reduce experimenter involvement, the equipment was changed so that the
recording of the data and the start of the runs were done automatically. On the other hand, the
experimenter did
not reduce his personal efforts to bring the subjects into a highly
motivated state.
Let us review the successive experimental steps: First, the subject was psychologically prepared,
seated with headphones in a comfortable chair, and the click volume was individually adjusted
as in the pilot test. The only remaining task for the experimenter was the pushing of a button to
initiate an automatic sequence of 10 test runs. When the button was pushed, a signal lamp was
turned off to let the subject know that a run had started and that he should listen for the next
click. When the run ended, the weak click was presented and a few seconds later the signal lamp
was turned on so that the subject could notice the end of the run even if he had missed the click.
At this time the counter reading (giving the length of the run) was automatically recorded on
paper punch tape for later computer evaluation. (In addition, the experimenter also recorded the
counter readings manually so that he could tell the subject his score immediately after a session.)
Ten seconds later the next run was automatically initiated and this was indicated to the subject
by the turn-off of the signal lamp. After 10 runs the automatic test cycle stopped, and a break of
typically 10 minutes was taken. Then the next 10 trials were completed in the same manner.
Randomness tests at the completion of the sessions, as in the pilot study, were consistent with
the theoretically expected average run
length of 64.
Table 1 (Column II) shows that in this confirmatory study the average run length in the test
sessions was again significantly reduced, from 64 to 55.34 steps, i.e., the average waiting time
for the next click was reduced from 6.40 to 5.53 seconds.
Table 2 (Column II) gives the shift in the distribution of the run lengths toward smaller values.
The PK Test with Pre-Recorded Targets
In the previous test a random sequence of hits (p = 1/64) and misses (q = 63/64) was produced
by the random generator during the test session. The subjects concentrated on the hits
(represented by weak clicks) and thereby appeared to exert a PK effect because the hits were
generated with increased frequency. In the following test the random sequence of hits and misses
which appeared in a test session with a subject was not generated during this session, but was
obtained by a replay of a sequence that was generated and recorded earlier (in the absence of both
subject and experimenter). This previously recorded sequence had been left untouched, i.e.,
nobody looked at the sequence until it was played back in the test session.
For the purpose of a comparison between "test runs" and "control
runs" only half of the prerecorded sequences was used as the target in a test session. The other
half was merely evaluated by a computer and thus not exposed to a subject's attention during a
test session.
Let us list the individual steps of the experiment in their proper time sequence: First the random
generator (with p = 1/64) was automatically activated (at the rate of 10 per second) in the absence
of subject and experimenter. The hits and misses were recorded as signals in the left and right
channels respectively on a stereo cassette recorder. After each hit (at the end of each "run"), the
number generation was interrupted for 10 seconds. During this time the number of trials made
since the previous hit (i.e., the length of the run) was recorded on paper punch tape. Thus we
Gave two independent records of the generator output: the magnetic tape and the paper punch
tape.
A first sequence of 60 runs generated in this manner was labeled A, and a second such sequence
was labeled B. One of these two sequences, A or B, was to serve as target in test sessions and the
other as a control. A total of 10 such pairs of 60-run sequences was used for the experiment.
It was decided in advance to use A or B as the target sequence if the last digit of the square root
of n as given by a 12-digit desk calculator was even or odd, with
n = 2 for the first (A, B) pair,
and n = 3, 5, 7 ... (successive primes) for the following nine (A, B) pairs used in the experiment.
Although this decision was predetermined, the experimenter became aware of it only after the
target generation when he calculated the square root of n.
After the first pair (A, B) of 60 runs was recorded, the first subject was selected. The test
sessions were held under the same conditions as those in the previous confirmatory test. The
only difference was that the targets came from the prerecorded magnetic tape rather than from
a momentarily operating random generator.
After three subjects had been tested in this manner, a second pair of 60 runs was recorded, the
target tape (A or B) was determined by calculating the square root of n with n = 3, the next three
subjects were tested, and so forth. The results of the experiment were computer evaluated from
the paper tape records. These records were found to be in agreement with the manual records of
the counter readings during the test sessions.
Table 1 (Columns III and IV) gives the main results. It is seen that the test runs showed a
significantly reduced run length (average 56.37), whereas the control runs showed no significant
deviation from the chance expectancy value of 64 for the run length. Note that the test and
control runs were generated under the same conditions, while the experimenter was unaware of
which runs would serve as test or control.
Table 2 (Columns III and IV) confirms these findings: the distribution of the run lengths in the
test runs is shifted toward shorter runs whereas the control runs show no abnormality in their
distribution.
A comparison between Columns II and III in Tables 1 and 2 suggests that the two
arrangements produced very similar PK effects: for the PK effect it did not matter whether the
targets were momentarily generated during the session or whether they were obtained by the
replay of a previously recorded random sequence.
THE SECOND EXPERIMENT: PK EFFECT WITH REPEATED
PRESENTATION OF PRE-RECORDED TARGETS
This experiment is a modification of a previously reported conventional PK experiment
(Schmidt, 1973) in which a fast binary random generator produced a sequence of "heads" and
"tails" at the rate of either 30 or 300 per second while the subject tried to enforce an increased
generation of heads. The subject received momentary feedback on his performance in either of
two ways. In part of the experiment the heads and tails were displayed as clicks in the right or
left ear respectively, and in the other part the deflection of a display needle from the center
position indicated the momentary scoring rate. The PK success rate appeared to be rather
independent of the type of display, but significantly dependent on the trial generation speed.
With p = 1/2, the average scoring rate at 30 trials per second was 51.6% whereas the scoring rate
at the generation speed of 300 per second was only 50.4%.
The main difference in the experiment to be reported now is that part of the targets were not
generated during the session, but came from a prerecorded sequence, so that the PK effect on
prerecorded targets could be further studied. In this experiment, however, an attempt was made
to explore more than the mere existence of PK with prerecorded targets, in the following three
respects:
1. During the test runs, trials with momentarily generated targets and with prerecorded
targets were alternated in order to make possible a more direct comparison between conventional
PK and PK on pre-recorded targets.
2. The pre-recorded targets were generated and recorded at the high speed of 300 per second,
whereas the replay in the test sessions occurred at the low speed of 10 per second. This
arrangement should test the prediction of a theoretical model (Schmidt, 1975) that the outcome
of the experiment would not depend on the method of target preparation, so that in particular the
high speed at the prerecording of the targets should not reduce the size of the PK effect.
3. In the first experiment described above the scores obtained with and without time
displacement were of the same magnitude. One might try to increase the scores under time
displacement conditions, however, by playing the recorded sequence back to the subject not just
once, but several times. Thus the subject would spend more effort on each individual event, i.e.,
each hit would lead to several rewarding success stimuli. The theoretical model suggests
specifically that N repeated attempts at the same target should add linearly (for small effects),
leading to an N-fold increase in the average deviation from the chance level provided the subject
is not aware that the same target is offered several times. If the subject knew this, his attitude
toward the task could change because he might consider a second PK attempt at a previously
presented target as useless. In the present experiment each prerecorded target was presented four
times to the subject, but in different sections of a run, and embedded between momentarily
generated targets, so that he was not likely to notice the repeated appearance of some targets.
During a test run the subject received feedback on the binary events, at the rate of 10 per second,
in two ways:
1. The deflection (to the right or left) of a display needle was proportional to the scoring rate
(heads minus tails)/(heads plus tails) averaged over the last 20 trials. Thus the momentary
position of the needle gave the average scoring rate during the preceding two seconds. The
subject's task was to move the randomly fluctuating needle mentally as far and as long as possible
to the left, corresponding to an increased generation rate of tails.
2. Coupled to the display needle was a variable frequency sound generator so that deflection
of the needle to the right or left was accompanied by an increase or decrease of the frequency.
The subject listened to the sound through headphones. His task was equivalent to lowering the
average frequency of the sound.
Both types of feedback were given simultaneously, but some of the subjects may have
concentrated more on one form or the other, and even closed their eyes in order to concentrate
better on the sound.
These test conditions seemed psychologically sufficiently similar to those in the earlier
experiment so that the experimenter felt confident in beginning the new experiment without a
further pilot study.
The Structure of the Test Run
The repeated presentation of the prerecorded targets and the mixing with the momentarily
generated targets was done as follows:
At the beginning of a test run, while the experimenter was talking with the subject, a sequence
of 128 binary random numbers was generated at the rate of 300 per second and stored in a
memory (TTL solid state memory 74200, built into a 128-bit circular shift register).
For the purposes of permanent record, the number of heads and tails in the sequence was
automatically printed on paper punch tape. At this stage neither the experimenter or the subject
had any knowledge about the generated sequence, and the subject did not even know that a target
sequence had been prepared.
Next, a sequence of 256 binary events was presented to the subject at the rate of 10 per second,
with the auditory plus visual feedback described above. The 128 even-numbered events in this
sequence were provided directly by the triggering of a binary random generator, but the 128
odd-numbered events came from the sequence stored in the memory. Thus the subject received
a mixture of prerecorded and momentarily generated targets.
During a subsequent intermission of about 15 seconds the subject looked at display counters for
the total score (number of heads and tails) in this part of the run, and the score was automatically
printed on paper punch tape. Then another sequence of 256 binary events was presented, based
on the same memory content. Thus the sequence of odd-numbered events was the same as
before, but the momentarily generated even-numbered events were new.
This procedure was repeated two more times so that the outcome of the run was determined by
512 momentarily generated targets and 128 prerecorded and four times presented targets.
At the end of the run the memory content was automatically read and the corresponding number
of heads and tails was again printed on paper punch tape. In this manner it could be verified that
the content of the memory had not changed during the session.
In order to verify the randomness of the generator (this randomness had been computer-tested
extensively in the past), five control runs were made before, and five control runs after each test
session. In each control run a sequence of 128 binary numbers was generated and recorded in
the memory, then the contents of the memory read and recorded. Thus the numbers for the
control runs were generated and recorded in the memory under the same conditions as the
corresponding numbers serving as prerecorded targets in the PK tests. The 200 control runs did
not show a bias (12,715 heads and 12,885 tails, CR = 1.1). As an additional precaution against
a constant generator bias, the target side of the generator outputs was alternated after each test
session.
The Test Subjects
The experiment was performed at the Mind Science Foundation in San Antonio during the spring
of 1975. Twenty subjects were selected from a group of 30 volunteers who had responded to a
newspaper advertisement or had contacted the laboratory independently. The
volunteers had the opportunity to try out several precognition and PK test devices and when they
expressed interest in the experiment now being reported they were either tested immediately or
asked to return on another day. Each subject contributed two runs of the type described. The two
runs were carried out in one session, but separated by a 15-minute intermission.
Results
Table 3
MAIN RESULTS OF THE SECOND EXPERIMENT
| Momentarily Generated Targets | Pre-Recorded and Four
Times Presented Targets |
Number of Trials | 20480 | 5120 |
Deviation | 167 | 151 |
Scoring Rate | 50.815 | 52.95 |
CR | 2.33 | 4.22 |
---|
t (19 df) | 1.83 | 3.97
|
---|
P (one-tailed) | .05 | .0005 |
The main results are given in Table 3. We are primarily interested
in three quantities: (a) the scoring rate on the momentarily generated targets, (b) the scoring rate
on the prerecorded and four times presented targets, and (c) the difference between these two
scoring
rates.
The average scoring rate of 50.815% on the 20,480 momentarily generated targets is marginally
significant. A t-test with the subject as the unit (19 df) or the evaluation of the CR value leads
to a (one-tailed) significance level of .05. The scoring rate is lower, but not to a statistically
significant degree, than the scoring rate of 51.6% obtained in a previously reported experiment
(Schmidt, 1973) carried out under similar conditions.
A higher scoring rate of 52.95% was obtained on the 5120 prerecorded and four times presented
targets. From the resulting CR = 4.22 or t = 3.97 (19 df, we obtain a significance level of .0005
(one-tailed).
In order to test whether the scoring rate of 52.95% for the pre-recorded targets is significantly
higher than the scoring rate of 50.815% on the momentarily generated targets, we compared for
each of the 20 subjects his scoring rate on the prerecorded targets with his scoring rate on the
momentarily generated targets. A t-test for the
difference between the two means gives t = 2.39 (19 df), which is significant at the .025 level
(one-tailed).
Thus our second experiment confirmed the existence of PK with prerecorded targets.
Furthermore, the data support the hypothesis that repeated feedback of the prerecorded data can
increase the scoring rate. A high scoring rate of nearly 53% on the prerecorded targets was
obtained in spite of the fact that they were recorded at high speeds which had previously
(Schmidt, 1973) led to much lower scoring rates. This may bias us toward the view that what
matters for the outcome of the test is not the physical-psychological conditions at the time of the
target generation, but rather the conditions at the time of replay during the test session.
THE THIRD EXPERIMENT: PK EFFECT ON RANDOM GENERATORS
WITH TWO DIFFERENT PROBABILITY-VALUES
In this experiment the action of PK on two random generators with hit probabilitites of 1/8 and
7/8 was compared. In order to present the targets from these two generators in a psychologically
balanced manner, the generators were activated during the test session in random sequence so
that the subject never knew whether the next target would be produced by one or the other
generator. The hits and misses were presented (in the first part of the experiment) as clicks
offered to the subject's left or right ear, no matter from which generator they originated.
So far this may appear to be a conventional PK test since the random generators were activated
during the test session. The random order in which the two generators were activated in the test
run, however, was determined by a prerecorded sequence of 64 "heads" and "tails." The sequence
was produced by a binary random generator with p = q = 1/2 prior to the overt PK test. This
sequence was played back during the test run so that each recorded head or tail triggered the
generation of one target by the "easy" generator (with p = 7/8) or the "difficult" generator (with
p = 1/8). Thus a greater number of heads in the sequence was favorable for a high total score,
and therefore the subject's motivation to succeed might affect the outcome of the prerecording.
In an attempt to increase such a possible PK effect on the prerecorded sequence, each sequence
was used in the test run four times in succession so that each head or tail caused four triggerings
of the easy or the difficult random generator.
With regard to the theoretical model (Schmidt, 1975) mentioned above, this experiment appears
interesting because the model predicts relationships among the scoring rates on the "easy" trials,
the "difficult" trials, and the prerecorded targets.
The Test Equipment
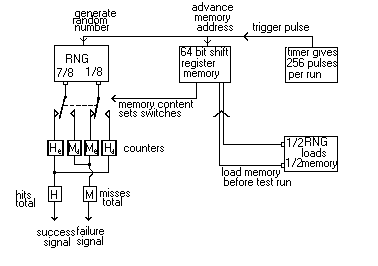
Fig.4. Block diagram for the setup in the third experiment.
During a test run a timer (see Figure 4) produced a sequence of 256
pulses at the rate of 10 per second. Each of these pulses triggered an unsymmetric binary random
generator so that a signal appeared at the left or right output channel of the generator with the
probabilities
of 7/8 and 1/8 respectively.
Which of these outputs was presented to the subject, and counted as a hit, depended on the
position of an electronic switch which is indicated in Figure 4 by a mechanical double-pole
double-throw switch. If this switch is in its left position (as drawn in the figure), a hit is
produced by a signal from the left generator output and we have an "easy" trial with the chance
hit probability of 7/8. For the other switch positions the hits come from the right channel with
a chance expectancy of 1/8 and we have a "difficult" trial.
Three counter pairs registered the total number of hits and misses, and also the hits and misses
for the easy and difficult trials separately. This redundant counting seemed desirable in this
experiment because the results were recorded only manually. The recording of all six counters
provided a safeguard against hidden recording errors.
Next, let us discuss the mechanism which set the electronic switch and thereby determined
whether a trial was an easy or a difficult one.
Prior to the overt PK test and while the experimenter was talking with the subject, a binary
symmetric random generator (with p = q = 1/2) was activated at the rate of 300 per second to
produce a sequence of 64 binary events. This sequence was stored in a 64-bit circular shift
register (built from the same elements as the memory in the second experiment, described above).
During the test run, with each timer pulse the next event in the shift register was read and,
depending on whether it was a "head" or a "tail," the selector switch for the easy and difficult
trials was set to the left or right position. In the 256 trials of the run the circular shift register was
read through four times. Thus a head or tail in the shift register produced four easy or difficult
trials respectively so that an increased number of heads in the prerecorded sequence was
favorable for success in the test.
The Subjects and the Test Arrangement
The experiment was performed during the fall of 1975 at the Mind Science Foundation in San
Antonio. The subjects were volunteers from among visitors to the laboratory. The pilot test
comprised 10 sessions with 10 different subjects. In the confirmatory study a total of 40 sessions
was carried out with 28 different subjects. Some of the subjects were permitted to take part in
more than one session (up to three sessions) because of a shortage of volunteers. This seemed
unobjectionable because we were interested primarily in the existence of an effect and relative
scoring rates under different physical conditions, but not in the question of how uniformly these
effects are distributed over a given subject population.
Each session involved four of the described test runs, separated by short intermissions. During
the intermissions the subject saw his total score and the experimenter recorded the readings of
all six counters. Furthermore, the sequence of 64 random events which determined the easy and
the difficult trials in the next run was automatically generated and stored in the shift register.
In the pilot test and for the first 20 sessions of the confirmatory study, feedback was given in the
form of clicks to the left ear (hits) or right ear (misses). In the last 20 sessions the hits and misses
were presented as low-pitched and high-pitched tones respectively.
Results
One objective of the experiment was the comparison of PK scores on "easy" trials, with a hit
probability of 7/8, and "difficult" trials, with a hit probability of only 1/8. These two types of
trials were offered in random sequence so that the subject was not consciously aware of whether
an easy or difficult trial was in progress.
Table 4
MAIN RESULTS OF THE THIRD EXPERIMENT
| Pre-Recorded Targets | Easy Trials | Difficult Trials |
| N_e | N_d | CR | theta | t |
H_e | M_e | CR | theta_e | t | H_d | M_d | CR | theta_d | t |
Pilot Test | 1341 | 1219 | 2.41 | 1.10 |
3.1 (9 df) | 4700 | 664 | 0.27 | 1.01 |
0.3 (9 df) | 580 | 4296 | -1.28 | 0.95 |
1.4 (9 df) |
Confirmation Session 1-20 | 2606 | 2514 | 1.29 | 1.04 |
0.6 (11df) | 9064 | 1360 | -1.69 | 0.95 |
1.3 (11 df) | 1345 | 8711 | 2.65 | 1.08 |
1.6 (11 df) |
Confirmation Session 21-40 | 2617 | 2503 | 1.59 | 1.05 |
1.2 (15df) | 9156 | 1312 | -0.10 | 1.00 |
0.0 (15 df) | 1245 | 8767 | -0.20 | 0.99 |
0.3 (15 df) |
Confirmation Total | 5223 | 5017 | 2.03 | 1.04 |
1.3 (27df) | 18220 | 2672 | -1.26 | 0.97 |
0.8 (27 df) | 2590 | 17478 | 1.74 | 1.04 |
1.0 (27 df) |
Note: N_e, N_d, number of "heads" and "tails" in the prerecorded sequences (p=q=1/2) Each
head or tall generates four "easy" or four "difficult" trials, respectively, in the test runs. H_e, M_e,
hits and misses on the easy trials (p hit = 7/8); H_d, M_d, hits
and misses on the difficult trials (p hit = 1/8).
Table 4 gives the main results for the pilot test and the confirmatory study. The results for the
first and second half of the confirmatory study are given individually because slightly different
types of feedback were used. In the first half the hits and misses were displayed as clicks to the
left and right ear respectively, whereas in the second half the hits and misses appeared as
low-pitched and
high-pitched tones.
As measure of the strength of the PK effect, the corresponding theta-values (Schmidt, 1975) are
given. These are defined as
theta_e=H_e/7M_e theta_d=7H_d/M_d
with
H_e,M_e: hits and misses in the easy trials
H_d,M_d: hits and misses in the difficult trials
Note that the definition of theta is always such that theta = 1 for chance
scoring and theta > 1 for a positive PK effect.
The theoretical model (Schmidt, 1975) predicts that, under somewhat idealized conditions (stable
and similar performance of all subjects), the two theta-values should be equal, theta_e=theta_d, for a
sufficiently long and statistically significant experiment. Unfortunately the CRs in Table 4
indicate that the results are not statistically significant so that the observettheta-value cannot be
considered close to the "real" theta-values. Therefore the results neither confirm nor falsify the
prediction of the model.
The other objective of the experiment was a study of the hypothesis that PK should also affect
the prerecorded sequences of heads and tails which determined the order of easy and difficult
trials in the test run. It was expected that an excess of heads (hits) should occur because each
head produced four easy trials, and an above-chance number of heads was thus highly
advantageous for the subject.
Table 4 gives the total numbers N_e for heads (producing easy trials) and N_d for tails (producing
difficult trials). The corresponding theta-value is defined as
theta' = N_e / N_d.
The pilot test seems to support the hypothesis of a PK effect. There are more heads than tails in
the sequences, with a significance level of .01 (CR = 2.41, and considering the 10 individual
sessions, t = 3.1
with 9 df).
The effect in the confirmatory study is reduced, however, and only marginally significant at the
.05 level (CR = 2.03, t = 1.3 with 27 df). Thus we have some indication of the existence of a PK
effect on the prerecorded targets, but the results are too weak to draw any more detailed
conclusions. In particular, we cannot check the prediction of the theoretical model that the
repeated use (four times) of the prerecorded targets should, for a sufficiently long and significant
experiment, lead to an increased theta-value, i.e., theta' = 4 theta_e = 4 theta_d.
One more analysis was made: Considering the values for theta_e, theta_d, theta' in the 40 sessions of the
confirmatory study, the correlation coefficients between these measures were determined. None
of these correlations were statistically significant.
DISCUSSION
The reported work indicates that PK effects can be obtained in tests with prerecorded targets.
The results suggest slightly more than the mere existence of such effects. The following points
appear
particularly interesting in this respect:
1. Whereas the main experiments reported above were conducted by one experimenter (the
present writer), significant results in
pilot tests were also obtained by two other experimenters. Particularly high PK scores on
prerecorded targets resulted from a test by Dr. E. F. Kelly with Mr. Bill Delmore as subject, who
had performed outstandingly in tests with several investigators. Thus it seems to be the subject
rather than the experimenter who is the PK source, and the effect does not appear to be restricted
to one experimenter.
2. In the first experiment conventional PK tests and corresponding tests with prerecorded
targets gave similar results, suggesting that the same basic PK mechanism was operating in both
situations.
3. In the first experiment test runs and control runs were prerecorded under the same
conditions so that not even the experimenter knew the difference. Nevertheless, only the test
recordings (which were presented as targets in a test session) showed anomalous scores. This
suggests that the subject's effort at the playback session was instrumental for the PK effect.
4. In the second experiment the prerecorded targets were generated at a very high speed
which had in earlier tests (Schmidt, 1973) led to a low scoring rate (50.4%). Nevertheless this
experiment, in which the targets were played back four times at the low rate of 10 per second,
produced a much higher scoring rate (52.95%). This again seems to favor the hypothesis that
what matters for the result is not the conditions at the time of target generation, but those at the
time when the targets are presented to the subject in the test session.
5. In the second experiment the scoring rate on the prerecorded and four times presented
targets was significantly higher than the scoring rate on the targets which were momentarily
generated and presented only once in a session. Thus it appears that repeated replay of the
prerecorded target sequence to one subject (or perhaps to several different subjects) can lead to
an increase in scoring rate.
6. In one of the pilot tests described above, the subject, Mr. Lalsingh Harribance, made his
PK efforts when he was alone at home, while he received immediate feedback from a tape
recorder. (The experiment was adequately safeguarded since the prerecorded targets to be used
in the evaluation of the results never left the laboratory; only a transcribed copy went out to the
subject.) In this manner it appears possible to channel PK effects into the laboratory from a
subject who is completely free to hold the test session wherever and whenever he wants.
With any interpretation of the results one has, of course, to be cautious for several reasons. First,
one might want to postpone serious discussion until we have more detailed experimental
information from several independent experimenters. Second, the failure of the third experiment
to give more than a marginally significant PK effect reminds us that we may still be overlooking
some vital factors which have a stronger effect on the test results than the variables we
are studying. And finally, a meaningful interpretation in this area of research, far outside our
intuitively accessible everyday experience, can be made only with reference to some theoretical
model: we have to devise logically consistent psi models and then see which of these models
agrees best with the experimental results.
One detailed model (Schmidt, 1975) and some more rudimentary models on the operation of PK
on prerecorded targets have been mentioned above in the introduction. The detailed model seems
consistent with the findings, and in particular with the addition effect in the second experiment.
On the other hand, the observed addition effect might also be explained in terms of the quantum
theoretical model in the sense that the first presentation of the targets, at the rate of 10 per
second, did not bring all the targets to the conscious awareness of the subject, so that repeated
presentation could improve the results. Further experiments which compare repeated feedback
and simple feedback at very slow rates might be able to distinguish between the two models, but
at present we cannot expect any of the few available models to be more than an incentive for
further experimentation.
REFERENCES
DEWITT, B. S. Resource letter IQM-1 on the interpretation of quantum mechanics. American
Journal of Physics, 1971, 39, 724-738.
SCHMIDT, H. PK tests with a high-speed random number
generator. Journal of Parapsychology, 1973, 37, 105-118.
SCHMIDT, H. Comparison of PK action on two different random number generators. Journal
of Parapsychology, 1974, 38, 47-55.
SCHMIDT, H. Toward a mathematical theory of psi.
Journal of the American Society for Psychical Research, 1975, 69, 301-319.
SCHMIDT, H., AND
PANTAS, L. Psi tests with internally different machines. Journal of Parapsychology, 1972, 36,
222-232.
STANFORD, R. G. An experimentally testable model for spontaneous psi events. II.
Psychokinetic events. Journal of the American Society for Psychical Research, 1974, 68,
321-356.